

Read the related blog on Diagnostic Reference Levels.
KV^5 6 EXPOSURE X RAYS HOW TO
That’s why it’s so important for the radiographer to understand how to read and utilize the exposure indicators. The reason for this increased risk is that we’ve lost the visual connection between the exposure and an image’s appearance. The potential for gross overexposure is one issue we encounter when a radiology department or clinic changes to a digital image receptor. So how does a radiographer know if a digital image is over- or under-exposed? Digital systems produce images with consistent density and contrast regardless of the exposure factors (See figure 2). However, with digital imaging devices, brightness and contrast are no longer linked to exposure factors. The density and contrast of the image on film is controlled by the kV, mAs and other exposure factors. You take your image, hold it up to the viewbox and say: “This image is too light” “This image is too dark” or, “This image is just right!” If you underexpose your image, it will be too light, and if you overexpose the image, it will be too dark (See figure 1).
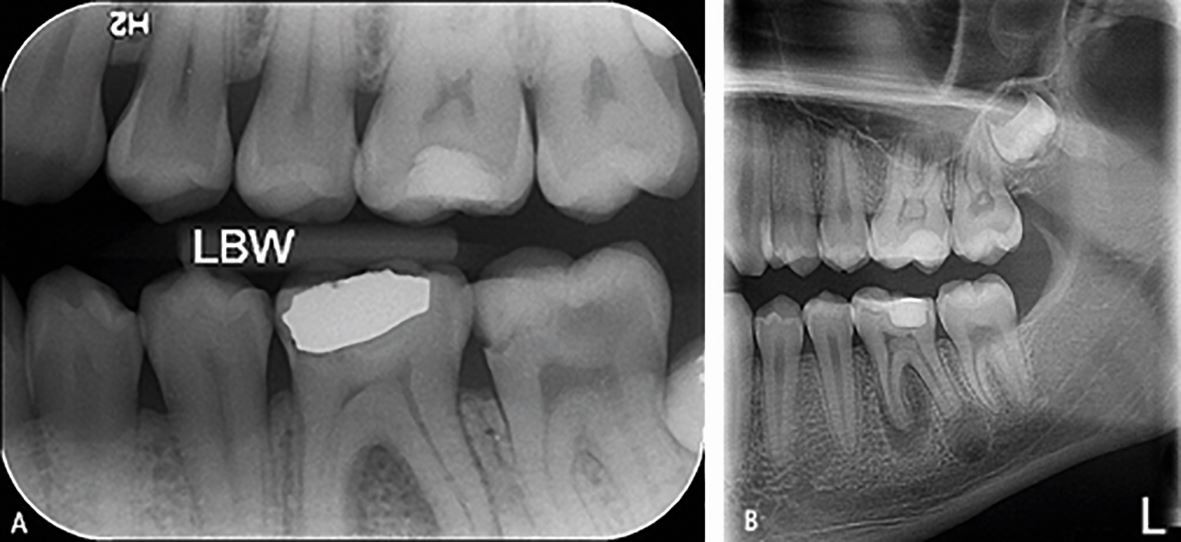
Imaging in a radiology film environment is much like playing Goldilocks and the Three Bears. These results are, with some restrictions, consistent with physical examinations and predictions concerning, for example, the assessment of the possible difference in effectiveness in causing strand breaks between mammography X rays and conventional (150-250 kV) X rays, indicating that differences in biological effects must arise through downstream processing of the damage.Reading Time: 5 minutes read Knowing how number is used is key to controlling exposure. It was found that the comet assay data did not indicate any difference in the initial radiation damage produced by 29 kV X rays relative to the reference radiation types, 220 kV X rays and the γ rays of 137 Cs and 60 Co, either for the total dose range or in the low-dose range. Data for the fraction of remaining damage were fitted to the simple function $F_=Al(t+A)$, where F d is the fraction of remaining damage, t is the time allowed for repair, and A (the only fit parameter) is the repair half-time. In addition, repair kinetics and residual DNA damage after exposure to a dose of 3 Gy were evaluated in three independent experiments for different repair times (10, 20, 30 and 60 min). The interexperimental variation and the influence of donor gender were negligible. Blood from only one male and one female was used. Irradiations with the different photon energies were performed simultaneously for each experiment to minimize interexperimental variation. Data from five independent experiments were transformed to natural logarithms and fitted using a multiple linear regression analysis. Fifty cells were analyzed per slide with a semiautomatic imaging system. The level of DNA damage, predominantly due to single-strand breaks, was computed as the Olive tail moment or percentage DNA in the tail for different air kerma doses (0.5, 0.75, 1, 1.5, 2 and 3 Gy). The aim was to measure human lymphocytes directly for DNA damage and subsequent repair kinetics induced by mammography 29 kV X rays relative to 220 kV X rays, 137 Cs γ rays and 60 Co γ rays. Experiments using the alkaline comet assay, which measures all single-strand breaks regardless of their origin, were performed to evaluate the biological effectiveness of photons with different energies in causing these breaks.
